Executive Preview
Naturally occurring pest control has intrigued scientists for thousands of years. The earliest known reference to diseases of insects is from the writings of Aristotle over 2,300 years ago. Throughout history, many scientists have expanded our knowledge of insect diseases and laid the foundation for the use of these diseases in pest management.
Modern scientists have expanded the knowledge about microorganisms that will control not only insects, mites, spiders, and nematodes but also bacterial, viral, and fungal diseases. These microbial pest controls include bacteria, fungi, viruses, nematodes, protozoa, and other microorganisms that impact populations or control pests.
While scientific advancements continue the exploration, is the best yet to come? The journey already has been long, yet fruitful; but new technologies create a high level of promise for the future.
The development and understanding of pathology and physiology of both insects and plants are fundamental to successful use of microbial pest control. This evolution faces many challenges in current crop production practices. Obstacles range from insect and weed resistance to product formulation, production scalability, shelf-life, application timing, and, of course, environmental conditions. Despite the lengthy scientific investigations into microbial pest controls, successful role models do exist.
Bt: Bacteria use as a Microbial Pest Control
Early development of the concept of microbial control was dominated by research on diseases of two important beneficial insects of the time, silkworm and honeybee. While working to control a problem, scientists realized a valuable use for the cause of the problem—a soil-dwelling bacteria known as Bacillus thuringiensis (Bt).
In 1901 a Japanese biologist, Shigetane Ishiwatari, was studying wilt disease in silkworms. His is the earliest record of the isolation of this Bt bacteria from an infected insect.
Between 1901 and 1938 researchers explored the mode of action that resulted in Bt’s insecticidal action. Bt produces proteinaceous crystals that are potent insect toxins. The conditions of how and when these toxins are produced were identified and the result was the realization of the insecticidal potential of Bt. Further basic research revealed that different strains of Bt produced toxins that were active against different groups of insects and each toxin had a very narrow host range. Some Bt strains produce insect toxins and others produce toxins effective against nematodes.
While manufacturers struggled with early formulations for commercial products, excellent performance was achieved with improved application and timing. The performance of microbial control products has continued to improve due to advancements in fermentation and harvesting procedures, identification of new and more active strains, and rigorous quality control. These have contributed to Bt products becoming the most developed of all microbial control products. In fact, Bt is the most commonly used biological pesticide worldwide. However, an apparently unlimited number of strains and extractions are yet to be discovered and developed.
Ongoing Discoveries
Currently 20 of the 50 Bt strains listed as active ingredients in the United States Environmental Protection Agency (EPA) product database are in registered pesticide products. These 20 strains represent five subspecies of Bt and a total of 346 active product registrations. After 100 years of research, new strains of Bt with novel pest activity are being identified regularly. There is no end in sight. Advanced technology currently allows the identification of new strains faster and more efficiently; the search for new strains through collections of wild populations is taking place at an accelerated rate.
In addition to the discovery of new strains, further characterization of known strains will continue to be identified. Better formulations and more efficient production methods will likely elevate many microbial control agents to commercial acceptability. Improvements in our understanding of the ecology of target insects and their pathogens, together with the optimization and use of genetic tools, will continue to contribute to the development and optimization of microbial control measures.
Further development will need to be supported and promoted by various stakeholder sectors to facilitate the market reach of these environmentally friendly solutions. The rapidly growing public interest in sustainable production practices is creating a highly receptive marketplace around the world.
AgriThority® is experienced at testing hundreds of different species of microbial control agents. Through our knowledge and expertise, we partner with technology developers throughout the entire development process. Our business, market and product development services, with regulatory support, covers the U.S. and many other countries around the world. Our expert network and services help move innovative and biological products across borders. This includes logistics for compliance with specific import and release permit requirements, as well as shipping and customs clearance management, protocol design, and implementation; all of which is supported by business and market development consultation. We are proud to play a role in transforming production practices toward sustainable and eco-friendly food systems, not only for the planet but also for all individuals who are part of it.
Read on to learn more about the evolution of microbial pest control agents.
View and download the PDF version of Evolution of Microbial Pest Control—The Best is Yet to Come.
Authors
Shaw has worked in agriculture for more than 40 years. An entomologist by training, Shaw most recently was Fiber Development Manager, Cotton Team, at Bayer CropScience, where he operated in the textile and apparel market globally with branded upland cotton fiber. His roles at Bayer CropScience and legacy companies Aventis CropScience, Rhone-Poulenc and Union Carbide spanned global product development, field development and technical services. He has managed technical support across broad-acre crops, vegetables, trees and vines, and specialty crops, including sugar cane and rice.
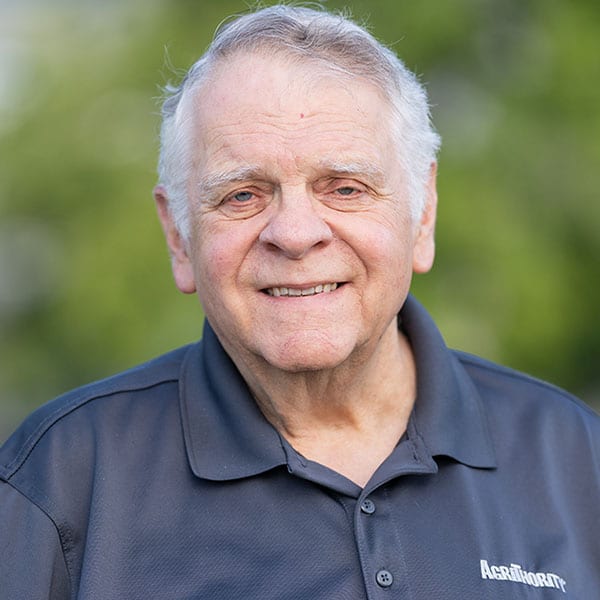
Eleonora Da Riva is a Master’s degreed entomologist and agronomist with project experience on “Spatial and temporal dynamic of Helicoverpa zea and Spodoptera frugiperda populations using pheromone traps in regions of Argentina.” As an AgriThority® Product Development Agronomist, LATAM, Eleonora applies her expertise in entomology, as well as biological and synthetic chemistries for crop protection.
Eleonora’s previous scientific roles expanded into product development team management following stints with AgIdea SA as entomology and microbiology services manager and laboratory scientist. Eleonora resides with her family in the small city of Funes near Rosario Argentina.
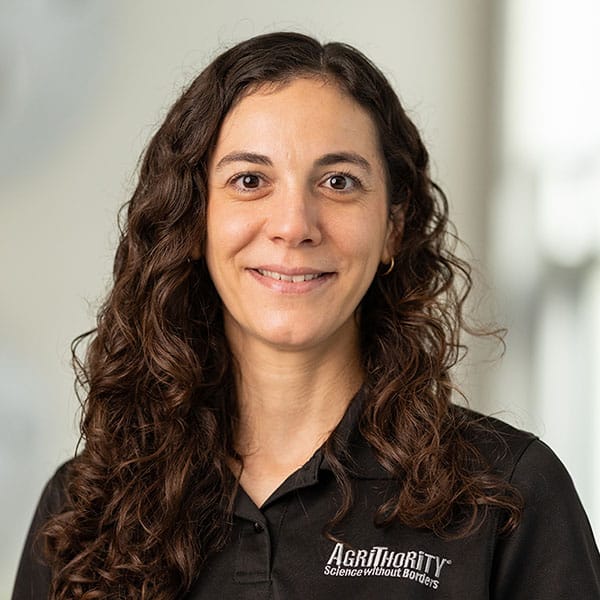
An expert biologist, Paula oversees client field trials across Europe to ensure protocols are followed and data will be quality. Previously, she coordinated a team of nine project managers conducting efficacy trials at AgIdea. Her responsibilities included evaluating the feasibility of protocols, working collaboratively with the PMs to ensure trials execution and monitoring, and doing the data and reports quality control. Paula also was Ecophysiology and modes of action Project Manager for AgIdea before taking the efficacy trial coordinator role where she executed efficacy trials for biostimulants, inoculants, fungicides and other new technologies. Prior to her work with AgIdea, Prieto was an ecophysiology researcher for University of Lleida in Spain.
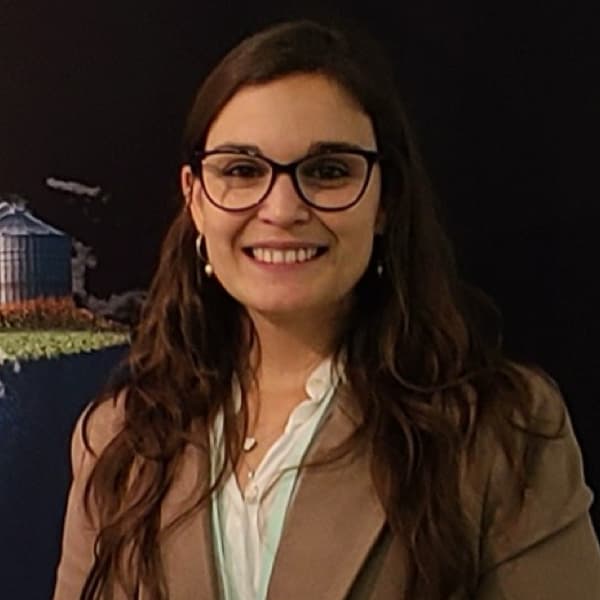
Evolution of Microbial Pest Control—The Best is Yet to Come
By Richard Shaw, Eleonora Da Riva, and Dr. Paula Prieto
Safe and effective pest management has long been a goal of crop protection. Civilizations have struggled to protect themselves and their food supplies from pest attacks for thousands of years. The earliest known reference to diseases of insects is from the writings of Aristotle over 2,300 years ago. Throughout history, many scientists have expanded their collective knowledge of insect diseases and laid the foundation for the use of these diseases as a pest management strategy.
The first attempt to use applied microbial control with bacteria was in the early 1900s when a bacterium, Coccobacillus acridiorum was applied for control of a grasshopper, Schistocerca americana in several Latin American countries with mixed results.
Over time, microbial pest control developed to include known microorganisms that will control insects, bacterial, viral, and fungal diseases, as well as mites, spiders, and nematodes. Microbial pest control is a subcategory of the practice of biological pest control, which is the use of naturally sourced pest control products. Microbial pest control organisms include bacteria, fungi, viruses, nematodes, protozoa, and any other microorganism that can be used to control pests. Biological control methods extend beyond microorganisms to include a variety of pest management strategies, such as the use of predatory insects, plant extracts, naturally occurring minerals, and synthetically produced versions of naturally occurring chemical compounds. Each category of biological control has its own fascinating history. However, this article will concentrate specifically on microbial pest control, exploring its evolution, current applications, and potential for future innovations in sustainable agriculture.
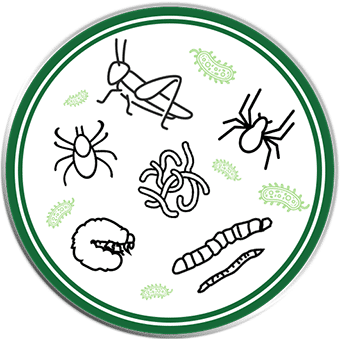
Bt: A Success Story Using Bacteria as a Microbial Pest Control
Early development of the concept of microbial control was dominated by research on diseases of two important beneficial insects of the time, silkworm and honeybee. While working to control a problem, scientists realized a valuable use for the cause of the problem—a soil-dwelling bacteria.
The earliest record of the isolation of Bacillus thuringiensis (Bt) from an infected insect was in 1901 by a Japanese biologist Shigetane Ishiwatari studying wilt disease in silkworms. He named it Bacillus sotto and it was later renamed Bacillus thuringiensis by a biologist, Ernst Berliner, who isolated it from a diseased Mediterranean flour moth in Germany. Around 1927, another German worker, Mattes, isolated the Bt strain discovered by Berliner and conducted field studies against the European corn borer.
Between 1901 and 1938 researchers explored the mode of action that identified the Bt insecticidal action. It was observed that Bt produces proteinaceous crystals that are potent insect toxins. The conditions of how and when these toxins are produced were identified and the result was the realization of the insecticidal potential of Bt. Further basic research revealed that different strains of Bt produced toxins that were active against different groups of insects and each toxin had a very narrow host range. Some Bt strains produce insect toxins while others produce toxins effective against nematodes. Bt strains toxic to other invertebrates are not yet developed as economic control measures.
The corn borer trials by Mattes showed promise and, by 1938 led to the development of the first commercial Bt insecticide in France. The first registration of Bt in the U.S. was in 1961 after many years of testing formulations that didn’t work. Although by the late 1960s when formulations were much improved, manufacturers still struggled with dry and dusty powders that were susceptible to lumping when mixing. But excellent performance was possible when the application and timing were right. Other advancements in the performance of microbial control products include improved fermentation and harvesting processes, the identification of new, more active strains, and rigorous quality control measures. While Bt products are the most developed among microbial control solutions, there still appears to be an almost limitless potential for discovering new strains and extractions.
Currently fifty Bt strains are listed as active ingredients in the United States Environmental Protection Agency (EPA) product database. Many of these are registered for use in genetically modified (GMO) crops or are not currently being used in a registered product. Twenty strains are used in registered pesticide products. These twenty strains represent five subspecies of Bt and a total of 346 active product registrations. After 100 years of research, new strains of Bt with novel pest activity are being identified regularly. There is no end in sight. Advanced technology currently allows the identification of new strains faster and more efficiently, and the search for new strains through collections of wild populations is accelerated.
In addition to the discovery of new strains, further characterization of known strains will continue. Better formulations and more efficient production methods will likely elevate many microbial control agents to commercial acceptability. Improvements in our understanding of the ecology of target insects and their pathogens, together with the optimization and use of genetic tools, will continue to contribute to the continued development and optimization of microbial control measures. Development will need to be supported and promoted by various sectors to facilitate the market reach of these products, where demand for more environmentally friendly solutions has increased, along with growing interest in organic and sustainable production practices.
Fundamental to the development of microbial control of insects has been the expansion of scientific understanding of pathology and physiology of insects and plants. One of the most noted pioneers and historians of insect pathology and biocontrol was Dr. Edward A. Steinhaus, credited by much of the scientific community as the father of insect pathology. In 1956, Steinhaus wrote “Microbial Control—The Emergence of an Idea” in Hilgardia, published by the California Agricultural Experiment Station. At the time of his death in 1969, he was working on a book on the history of insect pathology. His manuscripts were posthumously published in 1975 by the Ohio State University Press. The work is titled “Disease in a Minor Chord” and is one of the most complete accounts of the beginnings of the science of biological control, predominantly microbial control, of plant pests through the middle of the 20th century. Steinhaus’s publications were relied on for much of this summary.
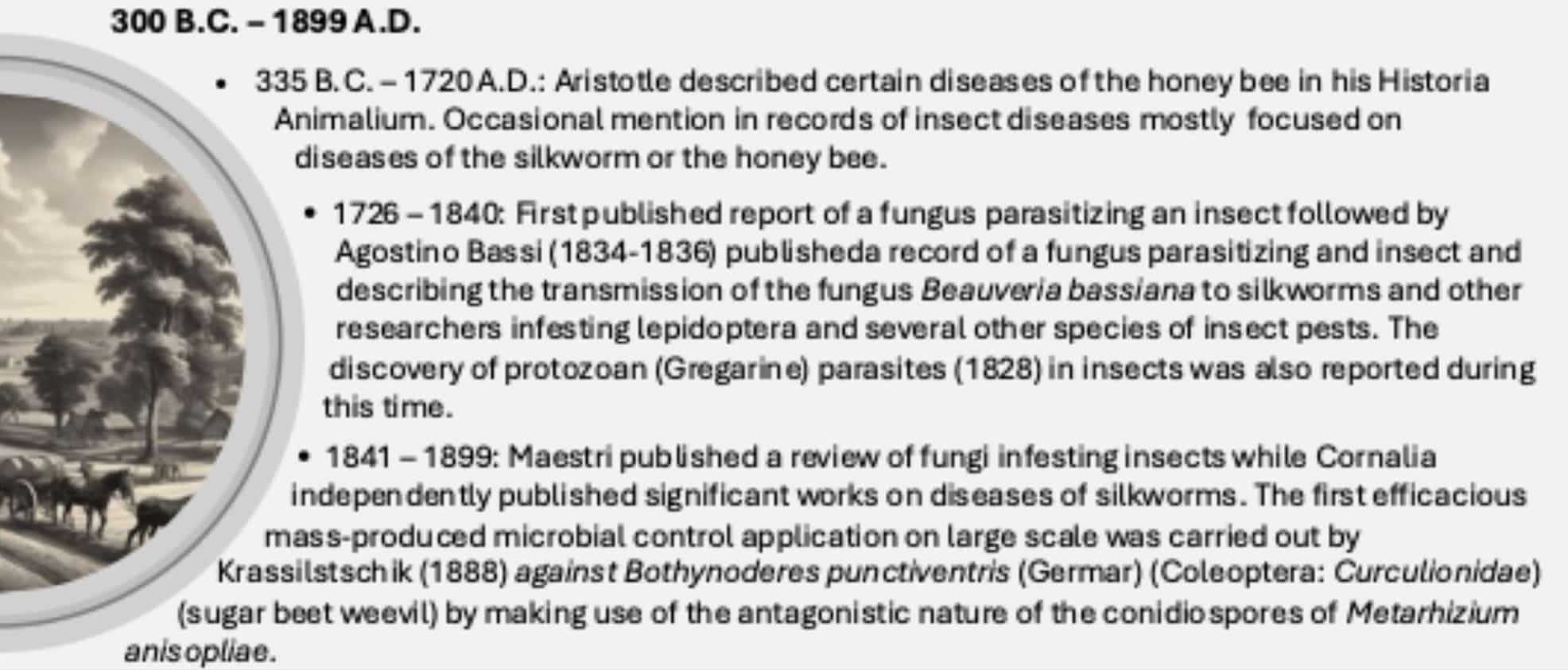
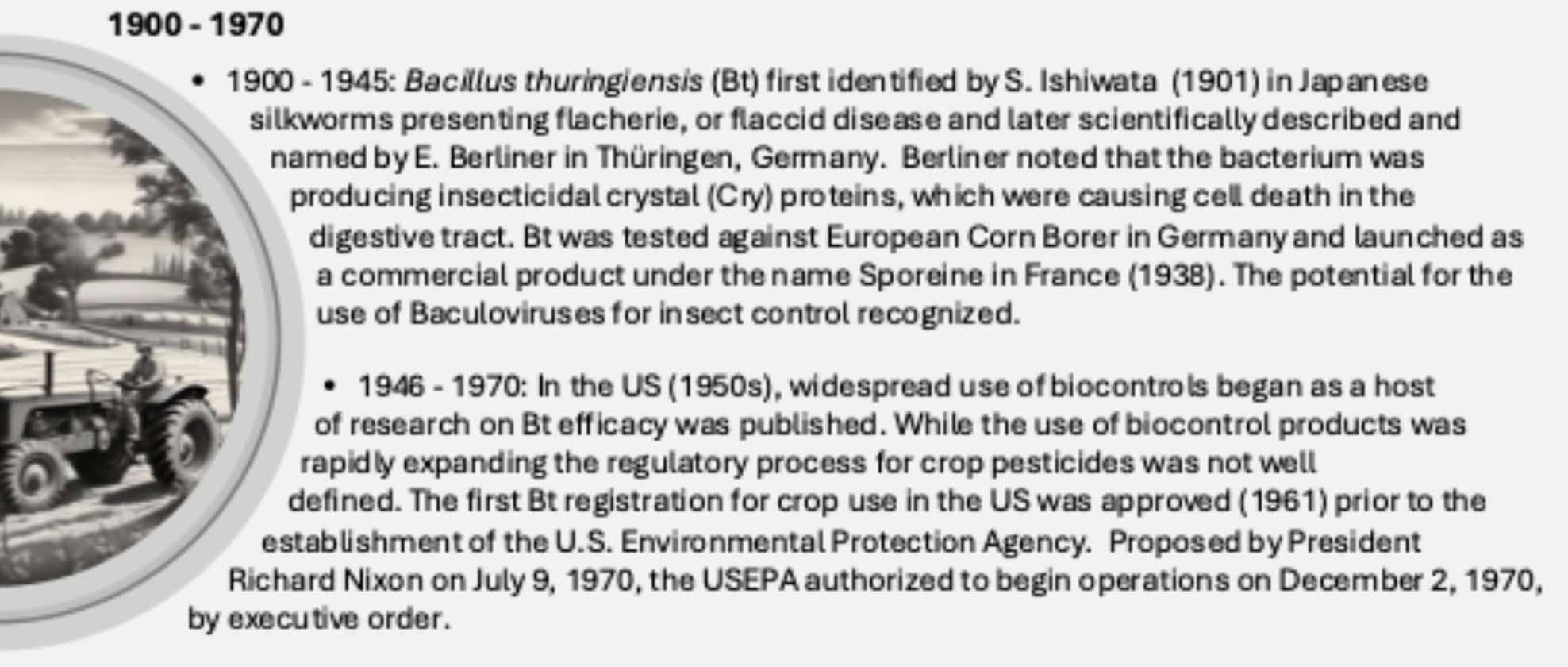
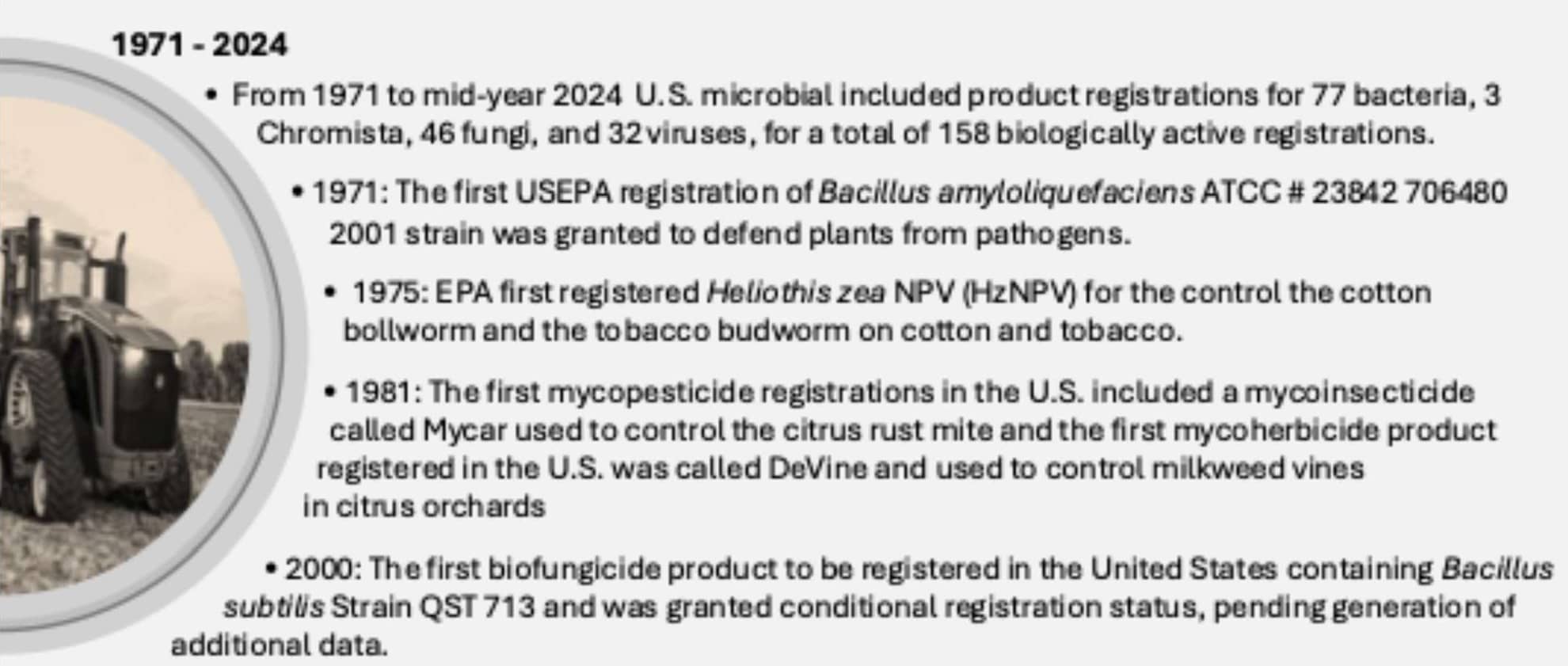
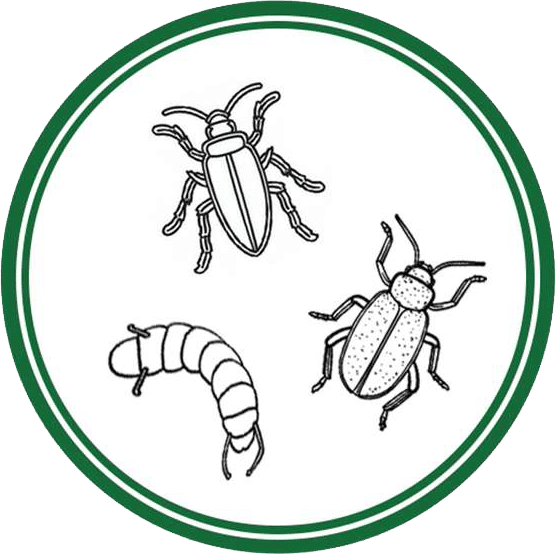
The Role of Fungi in Biological Control of Insects Pests
Throughout the latter part of the 19th century and the first half of the 20th century, many efforts to control important insect pests biologically were with fungi. These included attempts in Ukraine from 1879-1884, to control sugarbeet curculio (beet weevil), Cleonus punctiventris, with a fungus, Metarhizium anisoplia, and from 1888-1902, programs targeting chinch bugs, Blissus leucopterus, in the US Midwest with the fungus Beauveria bassiana.
Fungi are the most visible of insect diseases and in some cases the most virulent, causing massive die-off of insect populations. The positive and negative results with entomophagous fungi have affected the approach to all microbial control efforts. In recent years, an increased understanding of critical population densities for fungal outbreaks has made the manipulation of natural populations of fungal pathogens a critical part of some integrated pest management programs.
In 1835 an Italian bacteriologist, Augustino Bassi, was able to artificially transmit the fungus Beauveria bassiana to silkworms and other species. The fungus is common in soil and parasitizes many different species of insects. Currently, seven different strains of B. bassiana are registered by US EPA in numerous insecticide products.
A decade or more after the work of Bassi, the famous French microbiologist, Louis Pasteur, known primarily for his accomplishments in germ theory of disease, development of vaccines for rabies, cholera, and Anthrax, and the process of Pasteurization, also studied diseases affecting the silkworm industry in France. He is credited in Steinhaus’s writings with “initiating the real scientific development of insect pathology”. It is also observed that Pasteur’s work in insect pathology contributed to his historical advancements in the understanding and prevention of human disease.
The work of Bassi, Pasteur, and numerous other scientists across the globe intensified to define control methods for different pests by using microbial pathogens. Many insect/pathogen relationships were identified from these various works. More importantly, each effort revealed original information on host and pathogen interactions with the environment that led to the eventual commercial success of this approach to the management of insects and other pests of crops.
Interest in microbial control of insects grew rapidly in the United States as evidenced by the writings of J. L. Leconte. He was a famous and influential MD by training and entomologist by his life’s work. In 1875 he proposed an overhaul of economic entomology in the United States and included microbial control as an alternative that should be developed. This is believed to be the first formal, published proposal for the use of microbial pest control.
The Role of Fungi in Biological Weed Control
Plant pathogens were also introduced in 1970 as bioherbicides. Biological control of weeds involves the intentional use of living organisms, known as mycoherbicides, to reduce the vigor, reproductive capacity, density, or impact of weeds, positioning them as a potential alternative to chemical herbicides. Several selected microorganisms have been extensively studied, and many have been developed or are under development for commercial use.
Early mycoherbicides primarily relied on highly virulent fungal pathogens that infected the aerial parts of weeds, resulting in visible disease symptoms. Fungal genera such as Colletotrichum, Phoma, and Alternaria have received significant attention as promising microbial herbicide candidates. However, despite progress, little is known about host-pathogen interactions in natural plant communities, and even less is understood about weed-pathogen dynamics specifically.
Most of the existing research on classical biological weed control with plant pathogens is on the -Puccinia chondrillina. This relationship involves an apomictic plant population and an overly specific rust pathogen, which highlights the complexity of achieving broader applicability with bioherbicides.
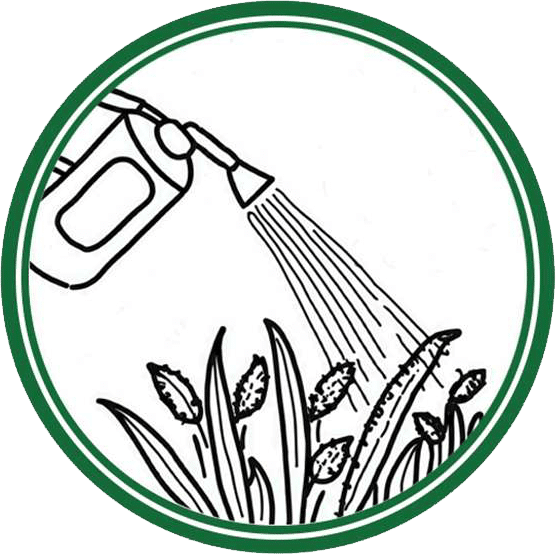
Successful incorporation of bioherbicides into conventional agriculture will only be achieved if they are able to effectively and consistently suppress multiple weeds of economic importance on a very large scale. Continued research and innovation are essential to unlocking the full potential of bioherbicides as a reliable and environmentally friendly solution for weed control. These also target specific weed species without affecting crops or beneficial plants, thus reducing the chance of resistance development and helping to manage weed populations more effectively over time.
Nematodes and Viruses to Control Insects and Weeds
In the decades following, other researchers discovered promising nematodes that attacked Japanese beetle. Use of live nematodes for insect control is exempt from registration by the U.S. EPA. According to an extension publication by University of Florida, six nematode species of the Genus Steinernema and five species of the nematode Genus Heterorhabditis are available for use on a wide range of insects attacking a wide range of crops. Target pests include numerous species of grubs, weevils, cutworms, armyworms, chinch bugs, thrips, mole crickets, and other soil infesting insect larvae.
Baculoviruses of two groups; nuclear polyhedrosis viruses (NPV) and granuloviruses (GV) were identified as pathogens of many lepidopterous pests of forests and agronomic crops. As of 2002, US EPA had registered occlusion bodies of five NPVs and two GVs as active ingredients for control of numerous lepidopteran pests. NPVs have been used in large-scale control programs in forestry for control of gypsy moth, pine sawfly, and other pests.
In select cases, viruses that affect undesirable plants have also been considered as bioherbicide candidates. Tobacco Mild Green Mosaic Tobamovirus for control of tropical soda apple (Solanum viarum) was investigated in Florida; Araujia Mosaic Virus for control of moth plant (Araujia hortorum) in New Zealand; and a virus resembling Tobacco Rattle Virus has also been proposed as a control agent for Impatien glandulifera, an invasive species of concern in central and western Europe.
The biological activities of viruses are very distinct from pathogenicity caused by bacteria or fungi and may present additional opportunities for biological weed control in some situations.
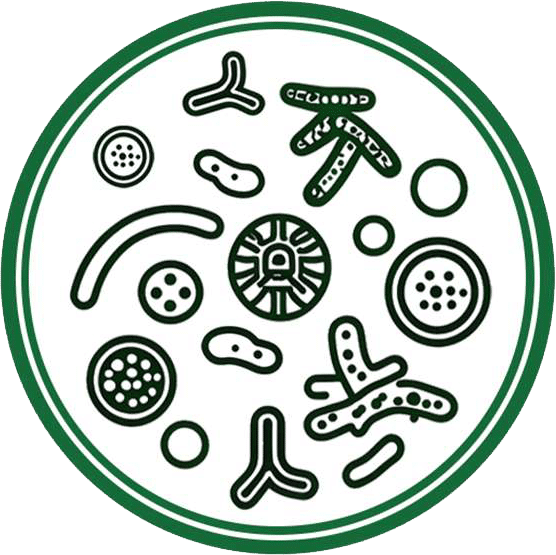
Microbials in Plant Disease Control
Additionally, the use of beneficial microorganisms as biological control agents against plant diseases is well documented. The most common agents include Trichoderma and bacteria from the genera Pseudomonas and Bacillus. These microorganisms employ various modes of action to suppress plant pathogens. These include competition for nutrients and space, the production of secondary metabolites and the induction of plant systemic resistance. Furthermore, they can target a wide range of pathogens, including fungi, bacteria, and viruses. Products containing these microorganisms are already available on the market.
Several bacteria have also been investigated as potential biological weed control agents with Pseudomonas fluorescens and Xanthomonas campestris drawing significant interest. Many strains of P. fluorescens, some of which are beneficial to plants whereas others are inhibitory. Notably, certain strains have been observed to selectively suppress the growth and germination of problematic grassy weeds like downy brome, showcasing the potential of microbial agents not just in disease control, but also in integrated weed management strategies.
A Growing Product Line
Microbial active ingredients registered in the EPA product database include 148 different microbes used in hundreds of registered pesticides. Chart 1 presents data from US EPA on the number of microbial active ingredients registered by EPA through October 2024. The rapid and extensive acceptance of microbial-originating plant incorporated protectants (PIP) through genetically modified organisms (GMO) has not slowed the continued development of new microbials for use as foliar, soil, and seed treatments. Sources available estimate the current 2024 global microbial pesticide market is between $1.37 Billion US and $2.68 Billion US. With projected annual growth forecast of five to six percent per year, this growth is approximately the rate of the entire global market for pesticides.
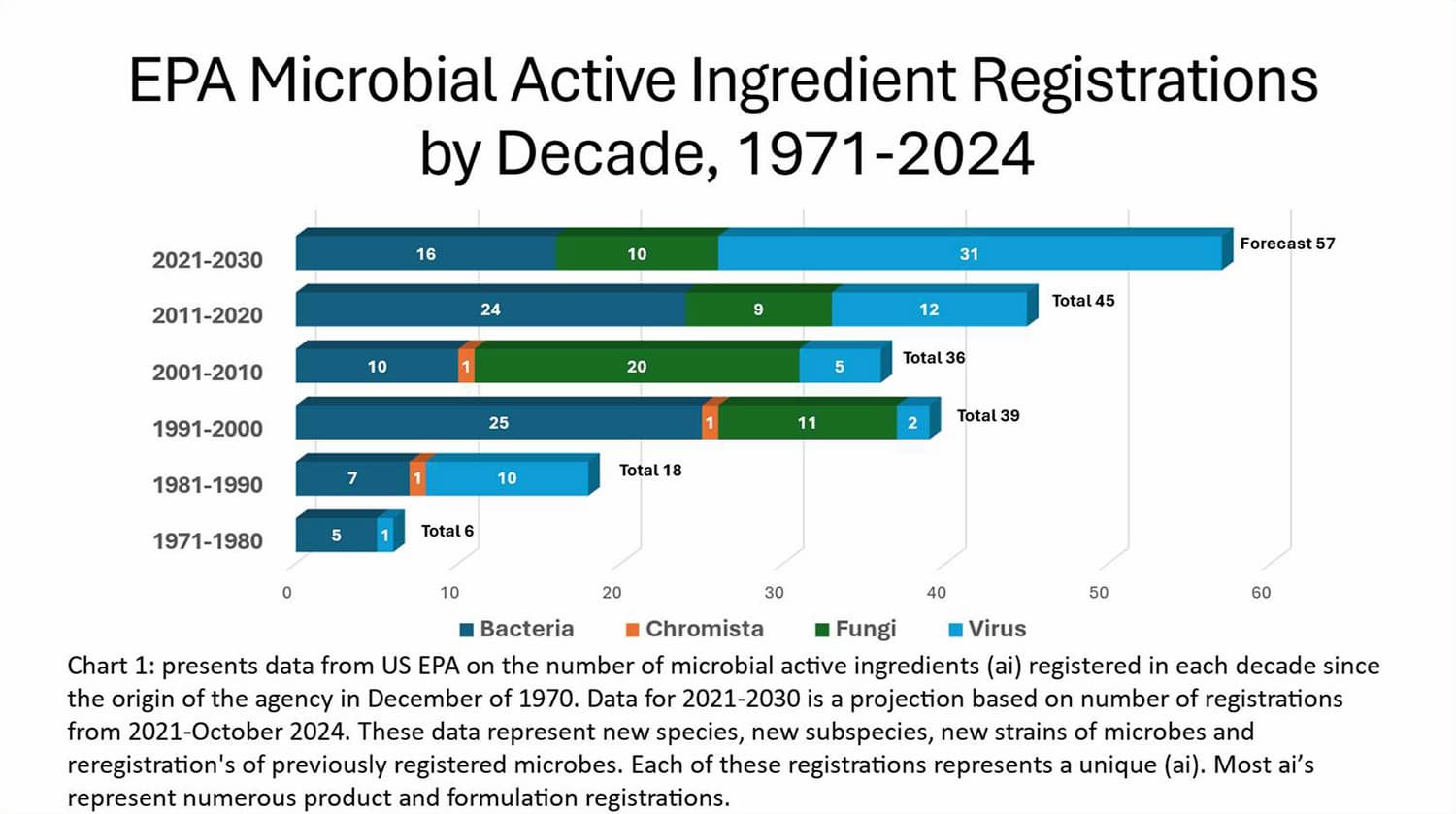
We have learned a lot since 1969 but as the father of insect pathology envisioned: we have barely scratched the surface. The statement applies to the science of microbial pest control. Many species of microbes have shown pesticidal potential that have not been explored in detail as much as Bt. As we learn more about the basic biology of these organisms, we will define how to use them effectively, and we will harness more of this field of science for practical application in pest management.
Scientific and technical challenges are just the beginning of problems in the evolution of microbial pest control. Challenges ranging from formulations, scalability in production, shelf-life, precise moment of application, environmental conditions, and most of all, the continued susceptibility of the target pests will be overcome to realize the full potential of this technology. Additionally, regulatory hurdles will likely be resolved as the complexity and lengthy timelines of the registration process are becoming a growing concern, impacting the level of investment that companies are willing to allocate toward developing new solutions.
As Dr. Edward A. Steinhaus, pioneer in biocontrol wrote in his final manuscript, “This book will be written with the conviction that most of what is to be historical in invertebrate pathology lies in the future, The best is yet to come”.
AgriThority is experienced at testing hundreds of different species of microbial control agents. With our knowledge and expertise, we partner with technology developers throughout their entire process. Our business, market, and product development services with regulatory support covers the US and many other countries around the world. Our expert network and services help move innovative and biological products across borders. This includes logistics for compliance with specific import and release permit requirements, as well as shipping and customs clearance management, protocol design and implementation, supported by business and market development consultation. We are proud to play a role in transforming production practices toward sustainable and eco-friendly food systems, not only for the planet but also for all individuals who are part of it.
Bibliography
Chattopadhyay, P., G. Banerjee, and S. Mukherjee, 2017, Recent trends of modern bacterial insecticides for pest control practice in integrated crop management system, 3 Biotech 7: 60
Harding DP, Raizada MN. 2015, Controlling weeds with fungi, bacteria and viruses: a review, Front Plant Sci. 2015 Aug 28;6:659. doi: 10.3389/fpls.2015.00659. PMID: 26379687; PMCID: PMC4551831.
LeConte, 1873, Hints for the Promotion of Economic Entomology, The American Naturalist Vol. 7, No. 12: 710-722 https://www.jstor.org/stable/2448012
Lord, J. C. 2005, From Metchnikoff to Monsanto and beyond: The path of microbial control. Publications from USDA_ARS/UNL Faculty. 363 https://digitalcommons.unl.edu/usdaarsfacpub/363/
Sanahuja, G., R. Banakar, R. M. Twyman, R. Capell, and P. Christou, 2011, Bacillus thuringiensis: a century of research, development and commercial applications, Plant Biotechnology Journal 9: 283-300 Plant Biotechnology Journal, 9: 283-300
Steinhaus, E. A. 1975, Disease in a Minor Chord. Ohio State University Press, Columbus: 488 pp. https://kb.osu.edu/bitstreams/2c1c47f9-c9a1-5763-bbf2-45cdc01a3980/download
Steinhaus, E. A. 1956, Microbial Control-The Emergence of an Idea, A Brief History of Insect Pathology Through the Nineteenth Century. Hilgardia 26: 107-160. http://hilgardia.ucanr.edu/Abstract/?a=hilg.v26n02p107
Tofangsazi, N. S. Arthurs, R. Giblin-Davis 2012, Featured Creatures, UF/IFAS University of Florida, Publication Number EENY-530. https://entnemdept.ufl.edu/creatures/nematode/entomopathogenic_nematode.htm
US EPA, 2024, Biopesticide Active Ingredients, US EPA. https://www.epa.gov/ingredients-used-pesticide-products/biopesticide-active-ingredients
US EPA, 2024, Pesticide Product and Label System, US EPA. https://ordspub.epa.gov/ords/pesticides/f?p=PPLS:1
Yongquan Li, Ziling Sun, Xiaofeng Zhuang, Ling Xu, Shifei Chen, Mingzhi Li, 2003, Research progress on microbial herbicides, Crop Protection, Volume 22, Issue 2, Pages 247-252, ISSN 0261-2194, https://doi.org/10.1016/S0261-2194(02)00189-8. (https://www.sciencedirect.com/science/article/pii/S0261219402001898)
Appendix 1
Time Interval
Key Events and Developments
335–322 BC
Aristotle described certain diseases of the honey bee in his Historia Animalium.
323 BC–1720
Occasional mention in records of insect diseases. Most were focused on diseases of the silkworm or the honey bee.
1721–1830
First published report of a fungus parasitizing an insect in 1726, Additional reports of fungi infecting insects and continued references to disease of honey bee and silkworm. First report of protozoan (Gregarine) parasites in insects in 1828.
1831–1860
Bassi, demonstrated the fungus Beauveria bassiana) was the cause of an infectious disease in silkworms, demonstrated that other insects were susceptible to the fungus, and was the first to suggest that fungi could be used to control insect pests. 1834-1836. Other researchers infected lepidoptera and several other species of insect pests. A review of fungi infesting insects was published and Maestri and Cornalia independently published significant works on diseases of silkworms in which they described the polyhedral bodies characteristic of NPV viral infection of insects in 1856. In 1858, a comprehensive review of the entomophagous fungi Cordyceps was published.
1861–1885
Housefly fungus was suggested as possible control method for mosquitoes, flies, and caterpillars in 1861. From 1865 to 1874, L. Pasteur conducted research on insect diseases caused by a protozoan, a virus, and soil fungi. He recommended use of microorganisms to control grape phylloxera. In 1875, J. L. Leconte, an MD by training with a lifetime dedication to entomology as well, suggested a focus on developing microbial controls, especially the use of fungi to control insects. In 1874, Metchnikoff and Krassilstschik experiment with M. anisopliae for sugar beet curculio, establish manufacturing plant for a microbial control agent. Introduce microbial control in Ukraine 1879-1884.
1886–1905
Attempts by S. A. Forbes, of Illinois, and F. H. Snow, of Kansas, to control the chinch bug by means of the fungus Beauveria globulifera (Speg.). Other aspects of insect pathology were studied by Forbes during this period. Snow and Forbes responsible for stimulating the interests of American entomologists in the possibilities inherent in microbial control methods. 1887-1898- In 1901, Bt was isolated from infected insect.
1906–1945
1927 Bt tested against European Corn Borer in Germany.
1938 Commercial Bt in France; Bt mode of action determined to be toxin production. In 1938 commercial Bt was offered for sale in France; Bt mode of action determined to be toxin production. In 1940s potential for use of Baculoviruses for insect control was recognized. 1906-1942 several attempts to organize area-wide projects in Florida to control several citrus pests with fungi met with mixed success.
1946–1970
This period is not well defined. A regulatory process for crop pesticides was not well defined. Many improved formulations were offered for sale in the US and globally. Research concentrated on process development to reduce cost, strain improvements to increase efficacy, and formulation development to improve mixing, handling, shelf-life, etc. efficacy was improved by six-to tenfold. Regulatory authority was distributed between several federal agencies and these records are not easily located.
1971–1980
In 1971, the US EPA was formed and the history of registrations in the US from 1071 to present are a reasonable representation of the advancement of microbial control technology.
US Registrations
Bacteria Chromista Fungi Virus
5 0 0 1
First Bt Kurstaki 3 strains | First Bacillus amyloliquefaciens | First NPV occlusion bodies of gypsy moth | First Agrobacterium radiobacter
1981–1990
US Registrations
Bacteria Chromista Fungi Virus
7 1 2 0
First Phytophthora palmivora | First Bt Israelensis 4 strains | First Bacillus lentimorbus | First Bt tenebrionis 2 strains | First Trichoderma polysporum registered | First T. viride strains
1991–2000
US Registrations
Bacteria Chromista Fungi Virus
25 1 11 2
First Bt aizawai strain | First Pseudomonas syringae | First P. fluorescens | First Bacillus subtilis | Additional Bt aizawai strain | First NPV occlusion bodies of Helicoverpa zea | Additional Bt kurstaki strain | Additional Streptomyces strain | First Metarhizium anisopliae strain | First Trichoderma harzianum 2 strains registered in the US.| Additional strains of Bt kurstaki | Additional strain of Pseudomonas syringae | First Ampelomyces quisquails isolate | First Bacillus popilliae | Additional strain of Bt kurstaki | First strain of Beauveria bassiana | Additional strain of Beauveria bassiana | First strain of Candida oleophilaisolate | Two additional Bt kurstaki strains | First Burkholderia cepacia strain | First Gliocladium virens strain | First Lagenidium giganteum | First Pseudomonas syringae strain | Additional Bt Israelensis strain | Additional strain of Bacillus amyloliquefaciens | First Gliocladium catenulatum strain | First Isaria fumosorosea Apopka strain | Additional Agrobacterium radiobacter strain | Additional Pseudomonas aureofaciens strain | First Bacillus sphaericus strain | Two additional strains of Bacillus subtilis| First Cydiapomonella granulovirus strain | First Trichoderma harzianum strain | additional strain of Trichoderma harzianum
2001–2010
US Registrations
Bacteria Chromista Fungi Virus
10 1 20 5
First Bacillus pumilus strain | First Coniothyrium minitrans strain | First Pseudomonas chloroaphis strain | First GV for Indian meal moth | Additional strain of Bt kurstaki | Two additional strains of Beauveria bassiana | First strain of Pseudozyma flocculosa | First Puccinia thlaspeos strain|, First NPV occlusion bodies of Anagrapha falcifera | First Aspergillus flavus strain | First Bacillus lichenformis strain | Additional strain of Metarhizium anisopliae | Registration of new strains of Aspergillus flavus | First strain of Bacillus pumilus | First strain Chonodrostereum purpureum | First strain Streptomyces lydicus | First strain of Alternaria destruens | First registration of bacteriophage active against Xanthomonas campestris | Pseudomonas syringae, First Paecilomyces lilacinus strain | Additional strain of Bt aizawai | Additional strain of Chonodrostereum purpureum. | First registration of Colletotrichum gloeosporioides strain | First registration of Pantoea agglomerans strain | Additional stain of Beauveria bassiana | First strain of Pythium oligandrum | First strain of Zucchini yellow mosaic virus | First Bacillus firmus strain | First Pasteurea usage strain | First Ulocladium oudemansil strain | Additional strain of Candida oleophila | First Trichoderma asperellum strain | First T. gamsii strain | First T. hamatumisolate strain
2011–2021
US Registrations
Bacteria Chromista Fungi Virus
24 0 9 12
First Chromobacterium substugae strain | Additional strain of Isaria fumosorosea | Additional four strain of Bacillus subtilis | First Aureobasidium pullulans strain | Additional Streptomyces acidiscables strain | Additional Pasteuria nishizaewae strain | First Pasteuria spp. for nematode control | First Trichoderma virens and Trichoderma harzianum mixture | Additional strain of BT kurstaki | Additional strain of Bacillus pumilus | First Bt galleriae strain | First NPV-U of Helicoverpa zea | Additional strain of Beauveria bassiana | Additional strain of Pseudomonas fluorescens | First NPV of Helicoverpa armigera | First MNPV of Spodoptera exigua | Additional strain of Bacillus subtilis | First Bacillus mycoides strain \ First strain of Papaya ringspot virus, Phlebiopsis gigantea | First MNPV of Spodoptera frugiperda | Additional Bt tenebrionis strain | Additional Bacillus amyloliquefaciens strain | Additional Bt kurstaki strain | Additional Bt Israelensis strain | First Wolbachia pipientis strain | Additional strains of Pseudomonas chloroaphis, Bacillus subtilis, and B. amyloliquefaciens | First Pepino mosaic virus strain | First cell walls of Saccharomyces cerevisiae | First Bacteriophage against Xanthomonas citri First Bacteriophage against Erwinia amylovora | Additional strains of Bacillus amyloliquefaciens, Bacillus lichenformis, Bacillus subtilis, and Beauveria bassiana | First MNPV for Autographa californica | First Bacteriophage against Xylella fastidiosa, and Clonostachys rosea | Additional Bacillus amyloliquefaciens strain | First NPV for Chrysodeixis includens, and Trichoderma atroviride | Additional strain of MNPV for Autographa californica.
2021–Oct 17, 2024
US Registrations
Bacteria Chromista Fungi Virus Amoeba
6 0 4 1 1
First Purpureocillium lilacinum strain | Reregistration of Beauveria bassiana strain GHA | First registration of Isaria fumosorosea strain | First Registration of Kosakonia cowanii strain | Reregistration of Beauveria bassiana strain ANT-03 | First registration of Willaertia magna strain | Reregistration of Bt Israelensis strain BMP 144 | First registration of GV for Cydia pomonella | Reregistration of Streptomyces strain K61 | Additional strain of Bacillus licheniformis | First Bacillus methylotrophicus strain | Additional Bacillus pumilus strain.